Wednesday 1 June 2011
Newton's First Law
In a previous chapter of study, the variety of ways by which motion can be described (words, graphs, diagrams, numbers, etc.) was discussed. In this unit (Newton's Laws of Motion), the ways in which motion can be explained will be discussed. Isaac Newton (a 17th century scientist) put forth a variety of laws that explain why objects move (or don't move) as they do. These three laws have become known as Newton's three laws of motion. The focus of Lesson 1 is Newton's first law of motion - sometimes referred to as the law of inertia.
Newton's first law of motion is often stated as
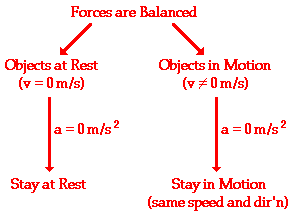
The behavior of all objects can be described by saying that objects tend to "keep on doing what they're doing" (unless acted upon by an unbalanced force). If at rest, they will continue in this same state of rest. If in motion with an eastward velocity of 5 m/s, they will continue in this same state of motion (5 m/s, East). If in motion with a leftward velocity of 2 m/s, they will continue in this same state of motion (2 m/s, left). The state of motion of an object is maintained as long as the object is not acted upon by an unbalanced force. All objects resist changes in their state of motion - they tend to "keep on doing what they're doing."
Suppose that you filled a baking dish to the rim with water and walked around an oval track making an attempt to complete a lap in the least amount of time. The water would have a tendency to spill from the container during specific locations on the track. In general the water spilled when:
The water spills whenever the state of motion of the container is changed. The water resisted this change in its own state of motion. The water tended to "keep on doing what it was doing." The container was moved from rest to a high speed at the starting line; the water remained at rest and spilled onto the table. The container was stopped near the finish line; the water kept moving and spilled over container's leading edge. The container was forced to move in a different direction to make it around a curve; the water kept moving in the same direction and spilled over its edge. The behavior of the water during the lap around the track can be explained by Newton's first law of motion.
Have you ever experienced inertia (resisting changes in your state of motion) in an automobile while it is braking to a stop? The force of the road on the locked wheels provides the unbalanced force to change the car's state of motion, yet there is no unbalanced force to change your own state of motion. Thus, you continue in motion, sliding along the seat in forward motion. A person in motion stays in motion with the same speed and in the same direction ... unless acted upon by the unbalanced force of a seat belt. Yes! Seat belts are used to provide safety for passengers whose motion is governed by Newton's laws. The seat belt provides the unbalanced force that brings you from a state of motion to a state of rest. Perhaps you could speculate what would occur when no seat belt is used.

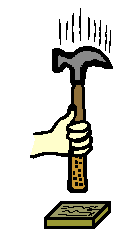
There are many more applications of Newton's first law of motion. Several applications are listed below. Perhaps you could think about the law of inertia and provide explanations for each application.

Newton's first law of motion is often stated as
An object at rest stays at rest and an object in motion stays in motion with the same speed and in the same direction unless acted upon by an unbalanced force.
There are two parts to this statement - one that predicts the behavior of stationary objects and the other that predicts the behavior of moving objects. The two parts are summarized in the following diagram.
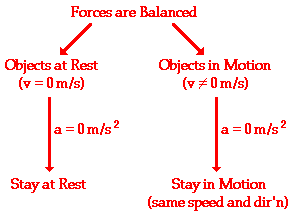
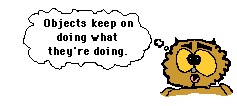
Suppose that you filled a baking dish to the rim with water and walked around an oval track making an attempt to complete a lap in the least amount of time. The water would have a tendency to spill from the container during specific locations on the track. In general the water spilled when:
- the container was at rest and you attempted to move it
- the container was in motion and you attempted to stop it
- the container was moving in one direction and you attempted to change its direction.
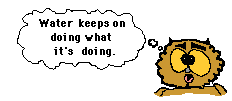
Everyday Applications of Newton's First Law
There are many applications of Newton's first law of motion. Consider some of your experiences in an automobile. Have you ever observed the behavior of coffee in a coffee cup filled to the rim while starting a car from rest or while bringing a car to rest from a state of motion? Coffee "keeps on doing what it is doing." When you accelerate a car from rest, the road provides an unbalanced force on the spinning wheels to push the car forward; yet the coffee (that was at rest) wants to stay at rest. While the car accelerates forward, the coffee remains in the same position; subsequently, the car accelerates out from under the coffee and the coffee spills in your lap. On the other hand, when braking from a state of motion the coffee continues forward with the same speed and in the same direction, ultimately hitting the windshield or the dash. Coffee in motion stays in motion.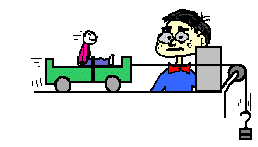

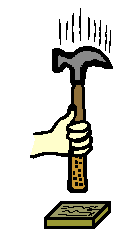
There are many more applications of Newton's first law of motion. Several applications are listed below. Perhaps you could think about the law of inertia and provide explanations for each application.
- Blood rushes from your head to your feet while quickly stopping when riding on a descending elevator.
- The head of a hammer can be tightened onto the wooden handle by banging the bottom of the handle against a hard surface.
- A brick is painlessly broken over the hand of a physics teacher by slamming it with a hammer. (CAUTION: do not attempt this at home!)
- To dislodge ketchup from the bottom of a ketchup bottle, it is often turned upside down and thrusted downward at high speeds and then abruptly halted.
- Headrests are placed in cars to prevent whiplash injuries during rear-end collisions.
- While riding a skateboard (or wagon or bicycle), you fly forward off the board when hitting a curb or rock or other object that abruptly halts the motion of the skateboard.

|
![]() |
Balanced and Unbalanced Forces
Newton's first law of motion has been frequently stated throughout this lesson.
But what exactly is meant by the phrase unbalanced force? What is an unbalanced force? In pursuit of an answer, we will first consider a physics book at rest on a tabletop. There are two forces acting upon the book. One force - the Earth's gravitational pull - exerts a downward force. The other force - the push of the table on the book (sometimes referred to as a normal force) - pushes upward on the book.
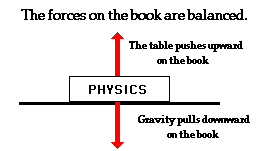
Since these two forces are of equal magnitude and in opposite directions, they balance each other. The book is said to be at equilibrium. There is no unbalanced force acting upon the book and thus the book maintains its state of motion. When all the forces acting upon an object balance each other, the object will be at equilibrium; it will not accelerate. (Note: diagrams such as the one above are known as free-body diagrams and will be discussed in detail in Lesson 2.)
Consider another example involving balanced forces - a person standing upon the ground. There are two forces acting upon the person. The force of gravity exerts a downward force. The floor of the floor exerts an upward force.
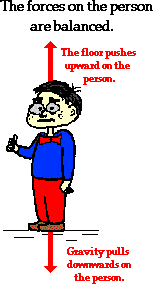
Since these two forces are of equal magnitude and in opposite directions, they balance each other. The person is at equilibrium. There is no unbalanced force acting upon the person and thus the person maintains its state of motion. (Note: diagrams such as the one above are known as free-body diagrams and will be discussed in detail in Lesson 2.)
Now consider a book sliding from left to right across a tabletop. Sometime in the prior history of the book, it may have been given a shove and set in motion from a rest position. Or perhaps it acquired its motion by sliding down an incline from an elevated position. Whatever the case, our focus is not upon the history of the book but rather upon the current situation of a book sliding to the right across a tabletop. The book is in motion and at the moment there is no one pushing it to the right. (Remember: a force is not needed to keep a moving object moving to the right.) The forces acting upon the book are shown below.
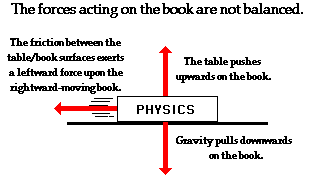
The force of gravity pulling downward and the force of the table pushing upwards on the book are of equal magnitude and opposite directions. These two forces balance each other. Yet there is no force present to balance the force of friction. As the book moves to the right, friction acts to the left to slow the book down. There is an unbalanced force; and as such, the book changes its state of motion. The book is not at equilibrium and subsequently accelerates. Unbalanced forces cause accelerations. In this case, the unbalanced force is directed opposite the book's motion and will cause it to slow down. (Note: diagrams such as the one above are known as free-body diagrams and will be discussed in detail in Lesson 2.)
To determine if the forces acting upon an object are balanced or unbalanced, an analysis must first be conducted to determine what forces are acting upon the object and in what direction. If two individual forces are of equal magnitude and opposite direction, then the forces are said to be balanced. An object is said to be acted upon by an unbalanced force only when there is an individual force that is not being balanced by a force of equal magnitude and in the opposite direction. Such analyses are discussed in Lesson 2 of this unit and applied in Lesson 3.
An object at rest stays at rest and an object in motion stays in motion with the same speed and in the same direction unless acted upon by an unbalanced force.
But what exactly is meant by the phrase unbalanced force? What is an unbalanced force? In pursuit of an answer, we will first consider a physics book at rest on a tabletop. There are two forces acting upon the book. One force - the Earth's gravitational pull - exerts a downward force. The other force - the push of the table on the book (sometimes referred to as a normal force) - pushes upward on the book.
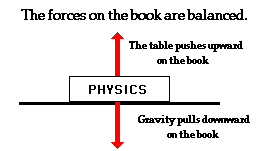
Consider another example involving balanced forces - a person standing upon the ground. There are two forces acting upon the person. The force of gravity exerts a downward force. The floor of the floor exerts an upward force.
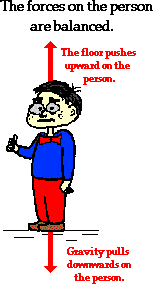
Now consider a book sliding from left to right across a tabletop. Sometime in the prior history of the book, it may have been given a shove and set in motion from a rest position. Or perhaps it acquired its motion by sliding down an incline from an elevated position. Whatever the case, our focus is not upon the history of the book but rather upon the current situation of a book sliding to the right across a tabletop. The book is in motion and at the moment there is no one pushing it to the right. (Remember: a force is not needed to keep a moving object moving to the right.) The forces acting upon the book are shown below.
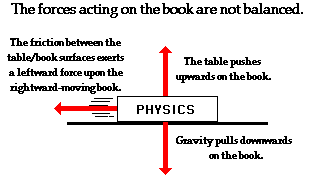
To determine if the forces acting upon an object are balanced or unbalanced, an analysis must first be conducted to determine what forces are acting upon the object and in what direction. If two individual forces are of equal magnitude and opposite direction, then the forces are said to be balanced. An object is said to be acted upon by an unbalanced force only when there is an individual force that is not being balanced by a force of equal magnitude and in the opposite direction. Such analyses are discussed in Lesson 2 of this unit and applied in Lesson 3.
State of Motion
Inertia is the tendency of an object to resist changes in its state of motion. But what is meant by the phrase state of motion? The state of motion of an object is defined by its velocity - the speed with a direction. Thus, inertia could be redefined as follows:
As learned in an earlier unit, an object that is not changing its velocity is said to have an acceleration of 0 m/s/s. Thus, we could provide an alternative means of defining inertia:
An object at rest has zero velocity - and (in the absence of an unbalanced force) will remain with a zero velocity. Such an object will not change its state of motion (i.e., velocity) unless acted upon by an unbalanced force. An object in motion with a velocity of 2 m/s, East will (in the absence of an unbalanced force) remain in motion with a velocity of 2 m/s, East. Such an object will not change its state of motion (i.e., velocity) unless acted upon by an unbalanced force. Objects resist changes in their velocity.Inertia: tendency of an object to resist changes in its velocity.
As learned in an earlier unit, an object that is not changing its velocity is said to have an acceleration of 0 m/s/s. Thus, we could provide an alternative means of defining inertia:
Inertia: tendency of an object to resist accelerations.
Inertia and Mass
Newton's first law of motion states that "An object at rest stays at rest and an object in motion stays in motion with the same speed and in the same direction unless acted upon by an unbalanced force." Objects tend to "keep on doing what they're doing." In fact, it is the natural tendency of objects to resist changes in their state of motion. This tendency to resist changes in their state of motion is described as inertia.
Galileo further observed that regardless of the angle at which the planes were oriented, the final height was almost always equal to the initial height. If the slope of the opposite incline were reduced, then the ball would roll a further distance in order to reach that original height.
Galileo's reasoning continued - if the opposite incline were elevated at nearly a 0-degree angle, then the ball would roll almost forever in an effort to reach the original height. And if the opposing incline was not even inclined at all (that is, if it were oriented along the horizontal), then ... an object in motion would continue in motion... .
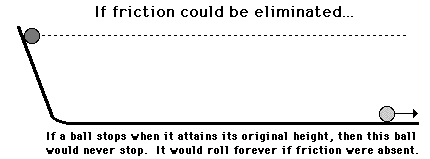
Isaac Newton built on Galileo's thoughts about motion. Newton's first law of motion declares that a force is not needed to keep an object in motion. Slide a book across a table and watch it slide to a rest position. The book in motion on the table top does not come to a rest position because of the absence of a force; rather it is the presence of a force - that force being the force of friction - that brings the book to a rest position. In the absence of a force of friction, the book would continue in motion with the same speed and direction - forever! (Or at least to the end of the table top.) A force is not required to keep a moving book in motion. In actuality, it is a force that brings the book to rest.
All objects resist changes in their state of motion. All objects have this tendency - they have inertia. But do some objects have more of a tendency to resist changes than others? Absolutely yes! The tendency of an object to resist changes in its state of motion varies with mass. Mass is that quantity that is solely dependent upon the inertia of an object. The more inertia that an object has, the more mass that it has. A more massive object has a greater tendency to resist changes in its state of motion.
Suppose that there are two seemingly identical bricks at rest on the physics lecture table. Yet one brick consists of mortar and the other brick consists of Styrofoam. Without lifting the bricks, how could you tell which brick was the Styrofoam brick? You could give the bricks an identical push in an effort to change their state of motion. The brick that offers the least resistance is the brick with the least inertia - and therefore the brick with the least mass (i.e., the Styrofoam brick).
A common physics demonstration relies on this principle that the more massive the object, the more that object resist changes in its state of motion. The demonstration goes as follows: several massive books are placed upon a teacher's head. A wooden board is placed on top of the books and a hammer is used to drive a nail into the board. Due to the large mass of the books, the force of the hammer is sufficiently resisted (inertia). This is demonstrated by the fact that the teacher does not feel the hammer blow. (Of course, this story may explain many of the observations that you previously have made concerning your "weird physics teacher.") A common variation of this demonstration involves breaking a brick over the teacher's hand using the swift blow of a hammer. The massive bricks resist the force and the hand is not hurt. (CAUTION: do not try these demonstrations at home.)
Newton's conception of inertia stood in direct opposition to more popular conceptions about motion. The dominant thought prior to Newton's day was that it was the natural tendency of objects to come to a rest position. Moving objects, so it was believed, would eventually stop moving; a force was necessary to keep an object moving. But if left to itself, a moving object would eventually come to rest and an object at rest would stay at rest; thus, the idea that dominated people's thinking for nearly 2000 years prior to Newton was that it was the natural tendency of all objects to assume a rest position.Inertia: the resistance an object has to a change in its state of motion.
Galileo and the Concept of Inertia
Galileo, a premier scientist in the seventeenth century, developed the concept of inertia. Galileo reasoned that moving objects eventually stop because of a force called friction. In experiments using a pair of inclined planes facing each other, Galileo observed that a ball would roll down one plane and up the opposite plane to approximately the same height. If smoother planes were used, the ball would roll up the opposite plane even closer to the original height. Galileo reasoned that any difference between initial and final heights was due to the presence of friction. Galileo postulated that if friction could be entirely eliminated, then the ball would reach exactly the same height.Galileo further observed that regardless of the angle at which the planes were oriented, the final height was almost always equal to the initial height. If the slope of the opposite incline were reduced, then the ball would roll a further distance in order to reach that original height.

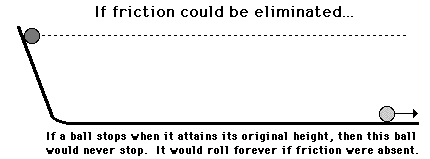
Forces Don't Keep Objects Moving
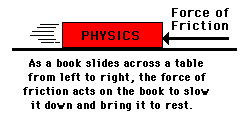
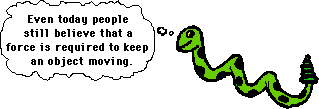
Mass as a Measure of the Amount of Inertia
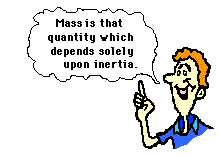
Suppose that there are two seemingly identical bricks at rest on the physics lecture table. Yet one brick consists of mortar and the other brick consists of Styrofoam. Without lifting the bricks, how could you tell which brick was the Styrofoam brick? You could give the bricks an identical push in an effort to change their state of motion. The brick that offers the least resistance is the brick with the least inertia - and therefore the brick with the least mass (i.e., the Styrofoam brick).
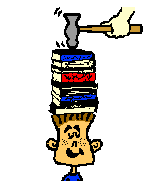
Subscribe to:
Posts (Atom)